Endotoxin Removal From Sterile Products By Rinsing Vs. Filtration
What is depyrogenation?
Depyrogenation is a process that removes pyrogens. The most prevalent and problematic pyrogens are the bacterial endotoxins found in the outer cell walls of gram-negative bacteria. Thus, depyrogenation is a process that will either destroy or remove bacterial endotoxins.
What are pyrogens?
Pyrogens are any molecules or substances that cause a feverous reaction when they enter the human body. Endogenous pyrogens (such as the cytokine interleukin-1) are found naturally within the human body. Endogenous pyrogens create a fever-producing reaction when naturally produced by the body. Exogenous pyrogens are molecules located outside of the body, such as endotoxins from gram-negative bacteria or pyrogenic prions. For context, prions are misfolded proteins that can transmit their misfolded shape onto regular versions of the same protein. Prions are of most concern for medical products and devices that interact with brain or spinal tissues. Exogenous pyrogens either provoke endogenous pyrogen production to create a fever within the body or activate the body’s toll-like receptors (TLRs) to trigger a fever. Clinically, the fever produced by endogenous cytokines is indistinguishable from fever produced by exogenous pyrogens such as lipopolysaccharide (LPS).
Why is depyrogenation needed?
Medical devices and parenteral products must be sterile and pyrogen-free. Products can accumulate pyrogens from raw materials or other parts of the manufacturing process. The best pyrogen removal or destruction process (known as depyrogenation) depends on the product. Standard depyrogenation methods are dry heat, rinsing, and filtration.
What is depyrogenation by rinsing?
Depyrogenation by rinsing is the process of destroying bacterial endotoxins through warm pressurized water exposure.
What is depyrogenation by filtration?
Depyrogenation by filtration removes endotoxins from solutions through adsorption and size exclusion.
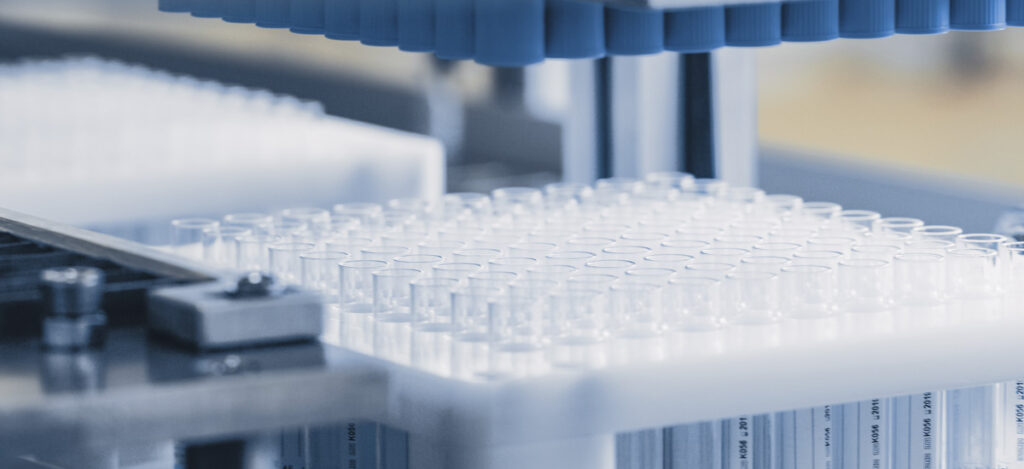
How is depyrogenation by rinsing performed?
Rinsing is the best means to remove bacterial endotoxins on closures (such as elastomeric stoppers), medical devices, and other materials that are not compatible with dry heat depyrogenation. The depyrogenation rinsing process is completed using high-purity water at temperatures above 60°C. Multiple rinse cycles remove pyrogens and ensure that the high-purity water is not contaminated with bacterial endotoxins during processing. Since rinse water purity is key to successful pyrogen removal, the rinse water quality must meet a minimum standard of less than 0.25 Endotoxin Units (EU) per milliliter. Poor water quality for rinsing may add pyrogens to medical devices and products at the same rate it removes them. Further, drying the items directly after rinsing is imperative to prevent microbial proliferation and post-rinsing endotoxin increases. Water for Injection (or other highly pure water) held for more than three or four hours below 55°C and above 8° should be considered a microbial contamination risk unless the water is sterilized. Solvents other than water, such as caustic alkali or detergents, can be used for depyrogenation by rinsing. However, these solvents may leave harmful residuals on products. Thus, if solvents other than water are used for rinsing, the depyrogenation validation must prove no toxic residuals are left on depyrogenated products.
For depyrogenation by rinsing, critical factors to define and control during processing are:
- Solvent description (including detergent description if used and the source of high-purity water)
- Solvent temperature
- Solvent pressure
- The flow rate of the solvent through the system
- Solvent recirculation (justification if used)
When removing endotoxins from a product via rinsing, the established endotoxin limit must not be exceeded by the sum of the endotoxin components of the active pharmaceutical ingredient (API) and any contributions by the excipients or other additives for parenteral products, pharmaceuticals, and other formulation products. For depyrogenation, the goal is often a 3-log reduction in recoverable endotoxin for the product. However, where a 3-log reduction is not required, inherent endotoxin burden and calculated endotoxin contamination risk can be used to justify depyrogenation processes that provide less than a 3-log reduction in pyrogens. The log reduction is calculated using the following formula:
Log10 reduction = (log10 recoverable activity) − (log10 residual activity)
How is depyrogenation by filtration performed?
Depyrogenation filtration processes are often performed on solutions containing proteins and peptides. Factors to consider when selecting your depyrogenation filters are the protein types in solution, protein concentration, electrolyte concentration, pH buffer system, molecular weight and isoelectric point (pI) of the protein, filtration flow rate, and protein aggregation potential. Depyrogenation of liquids may be accomplished employing filtration through more than one filter type. The primary filter types used for depyrogenation are described below. These filters are specific to depyrogenation and not intended for microorganism removal. However, some depyrogenation filters can retain certain microorganisms.
The following six filtration types are used for sterile product depyrogenation:
#1: Microporous Membrane Filters
Microporous membranes with pore size or retention ratings between 1.0 and 0.1 microns (μm) are effective at removing intact bacteria via size exclusion. Filtering freshly prepared solutions with microporous membranes can remove microbes, stop bacterial proliferation, and prevent endotoxin formation. Endotoxins themselves are much smaller than microporous membrane filter pores. Indeed, endotoxins are gram-negative bacterial cell wall fragments often less than 0.025 μm in size. However, endotoxins are negatively charged and can be removed through adsorption with positively charged membranes.
Endotoxin adsorption will occur with positively charged membranes until the membrane is saturated. Thus, it is important to change filters before saturation, or remaining endotoxins will pass through the filter. How well a positively charged membrane adsorbs the endotoxins is impacted by flow rate, pH, and any surface properties of the membrane. For example, if the flow rate is too fast for negative and positive charge interactions to occur, the endotoxins will not be extracted from the solution. As an alternative to positive charge adsorption, endotoxins have some adsorption affinity to hydrophobic membranes.
#2: Reverse Osmosis Membranes
Reverse osmosis (RO) membranes are the tightest size separation filters and are capable of removing dissolved salts and sugars from water. Essentially everything, including pyrogens, is extracted via size exclusion. RO membranes filter best at high pressure (200–1000 psi), which allows the filter to overcome osmotic pressure. RO membrane filter ratings are measured by retaining or removing marker salts such as sodium chloride or magnesium sulfate. RO membranes can be ultrafiltration system components (see #3 below) or single polymeric membranes. RO systems are often paired with downstream ultraviolet light if you wish to filter pyrogens and microbial contaminants. The primary risk for microbial contamination is that RO filters are used at ambient temperatures, capable of allowing the growth of any microbiological contamination.
#3: Ultrafiltration Membranes
Ultrafiltration (UF) membranes have pore sizes between one and one hundred nanometers. These filters are traditionally rated by molecular weight cut-off (MWCO) and are made of polymeric porous structures. Since the endotoxin subunit, LPS, is about 10–20 kilo Daltons (kDa), membranes of 6–10 kDa MWCO are used for size exclusion depyrogenation. However, LPS is commonly in aggregated forms weighing 300 to 1000 kDa so that endotoxin can be successfully removed by MWCO membranes of 30–100 kDa. UF depyrogenation capability can be boosted using the adsorptive capabilities of hydrophobic membranes. Overall, UF is not recommended for depyrogenation of solutions containing large proteins but is highly effective at removing endotoxins for small molecule drugs, buffers, electrolytes, antibiotics, and antifungal agents.
#4: Charge-Modified Depth Filters
Depth filters function through size exclusion, via sieving or entrapment, and adsorption, via electrokinetic or hydrophobic interactions. A filter’s ability to exclude particles by size alters based on the liquid’s flow path (distance) through the membranes. Electrokinetic adsorption occurs with various resin binders and filter aids that give the filter a positive charge (also known as a positive zeta potential). Like with microporous filters, positive zeta potentials allow filters to remove negatively charged endotoxins that are smaller than the rating of the filter’s pore sizes. Cellulose-based depth filters use filter aids to improve particle retention and product flow. Diatomaceous earth, perlite, carbon, and silica-based materials are examples of filter aids. The cartridges and capsules of depth filters are made of polypropylene, elastomers, or other plastics. Depth filter efficiency depends on many factors like the filtration materials (e.g., cellulose, filter aids, binding resins), product fluid characteristics (e.g., viscosity, dirt load, cell debris quantity, temperature), and fluid particle characteristics (e.g., hardness, abundance, size, aggregation potential, colloidal activity).
Charge-modified depth filters are advantageous in some situations than positively charged membrane filters because they allow less endotoxin to pass through the filtered product when the filter reaches its endotoxin filtration capacity. Further, charge-modified depth filters are extremely effective with a 4-log or 5-log reduction in endotoxin removal from products. This 4-log or 5-log reduction also applies to removing DNA fragments, host cell protein, and viruses. However, cellulose-based depth filters often contain glucans that are reactive to the limulus amebocyte lysate (LAL) used in bacterial endotoxin tests. Thus, products filtered with cellulose-based depth filters will require glucan removal or inactivation to prevent a false-positive bacterial endotoxin test result. In tricky filtrations, such as colloids, bacteria, or certain endotoxin levels, cost savings can be gained by using an appropriate depth filter.
#5: Activated Carbon Depth Filters
Activated carbon is a filter adsorbent that binds colors, odors, bacterial endotoxins, and nucleic acids. An organic material, activated carbon, has a microstructure with millions of pores. These pores give activated carbon its highly adsorptive properties and provide a far greater surface area for endotoxins to interact with than polymeric microporous structures. Activated carbon is only part of a depth filter and is often activated by steam or acid chemical treatment. As one of the best endotoxin removal agents, activated carbon can reduce endotoxin from liquids by a 4-log or 5-log reduction. However, active carbon provides nonspecific adsorption and can remove other important elements or molecules from a liquid during filtration.
#6: Membrane Adsorbers
When the therapeutic protein in solution has the same molecular weight range as endotoxins (10–20 kDa), ultrafiltration will not work to separate endotoxins from the therapeutic protein formulation. Charge-modified membrane adsorbers are used instead of ultrafiltration in this case. Membrane adsorbers are a manufacturing alternative to ion-exchange chromatography and are used to counter the handling and usage issues (such as slow filtration speed) utilizing ion-exchange chromatography would have for a scaled-up manufacturing process. In membrane adsorbers, ligands attached to the filter membrane’s surface provide endotoxin removal. Two primary strategies are used for product pyrogen removal with these filters: 1) using a strongly basic anion exchanger (type Q) or 2) using a strongly acidic ion exchanger (type S). In type Q membrane adsorbers, the basic exchanger (quaternary amine) is in a buffer with lower pH than the isoelectric point of the therapeutic protein. Type Q adsorbers bind endotoxin to the charged membrane surface and allow the therapeutic protein to pass through the membrane. In contrast, type S adsorbers use an acidic ion exchanger (also in a buffer with a pH lower than the isoelectric point of the therapeutic protein) to bind the therapeutic protein and allow the endotoxin to pass through the membrane. After initial type S filtration, the bound protein can be eluted using special buffers. Type S filters are commonly used to process monoclonal antibodies and recombinant proteins. Type S and Type Q filters are highly effective and provide a 4-log or greater reduction in endotoxins from products. Mixed-mode membrane adsorbers can be used as an alternative to type Q and type S membrane adsorbers. These membrane adsorbers only provide endotoxin removal on 3-log or 4-log reduction scale. However, mixed-mode membranes are superior at depyrogenation of protein solutions with high salt concentrations (e.g., 100–500 mM) compared to Q-type and S-type filters. In mixed-mode membranes, anionic and hydrophobic chemistries are used to bind endotoxins to the membrane surface. At the same time, salt and pH balancing flushes harness the power of charge repulsion to allow therapeutic proteins to flow through the membrane.
When to use depyrogenation by rinsing?
If medical devices or products cannot undergo the high temperatures of dry heat depyrogenation, depyrogenation by rinsing is recommended.
When to use depyrogenation by filtration?
Depyrogenation filtration processes are for liquids (e.g., solutions, suspensions, emulsions, and the like). Nearly all solutions containing proteins and peptides have their pyrogens removed by filtration.
Summary
Depyrogenation is a process that removes pyrogens. Testing for pyrogens is an imperative safety metric for regulatory approval of a medical device or product. The most prevalent and problematic pyrogens are the bacterial endotoxins found in the outer cell walls of gram-negative bacteria. Depyrogenation by rinsing is the process of removing bacterial endotoxins through exposure to warm, pressurized water. Alternatively, depyrogenation by filtration removes endotoxins from solutions through adsorption and size exclusion. Rinsing is a common depyrogenation method for medical products and other materials that cannot undergo dry heat depyrogenation. Depyrogenation filtration processes are often performed on solutions, suspensions, emulsions, and products containing proteins and peptides. All in all, ensure you choose a contract testing organization that can provide appropriate bacterial endotoxin testing and depyrogenation for your product needs.
Ethide Labs is a contract testing organization specializing in Bacterial Endotoxin Testing and Sterilization Validations. Ethide Labs also offers Microbiology Testing, Bioburden Testing, Sterility Testing, EO Residual Testing, Cytotoxicity Testing, Environmental Monitoring & Package Integrity Testing services for medical device companies and allied industries. Ethide is an ISO 13485 certified facility.
References
Review: Infection, fever, and exogenous and endogenous pyrogens: some concepts have changed. Innate Immunity. August 1, 2004.
Galanos C. and Freudenberg M. A. Bacterial endotoxins: biological properties and mechanisms of action. Mediators of Inflammation. 1993; 2(7): S11–S16.
Michael J. Akers. Sterile Drug Products Formulation, Packaging, Manufacture, and Quality. Drugs and the Pharmaceutical Sciences. Informa Healthcare. 2010.
United States Pharmacopeial Convention. <85> Bacterial Endotoxins Test. Rockville, MD, USA. 2021. (USPC <85>).
United States Pharmacopeial Convention. <1115> Bioburden Control of Non-Sterile Drug Substances and Products. Rockville, MD, USA. 2021. (USPC <1115>).
United States Pharmacopeial Convention. <1116> Microbiological Control & Monitoring of Aseptic Processing Environments. Rockville, MD, USA. 2021. (USPC <1116>).
United States Pharmacopeial Convention. <1228.3> Depyrogenation By Filtration. Rockville, MD, USA. 2021. (USPC <1228.3>).
United States Pharmacopeial Convention. <1228.4> Depyrogenation By Rinsing. Rockville, MD, USA. 2021. (USPC <1228.4>).
Share this in your social networks